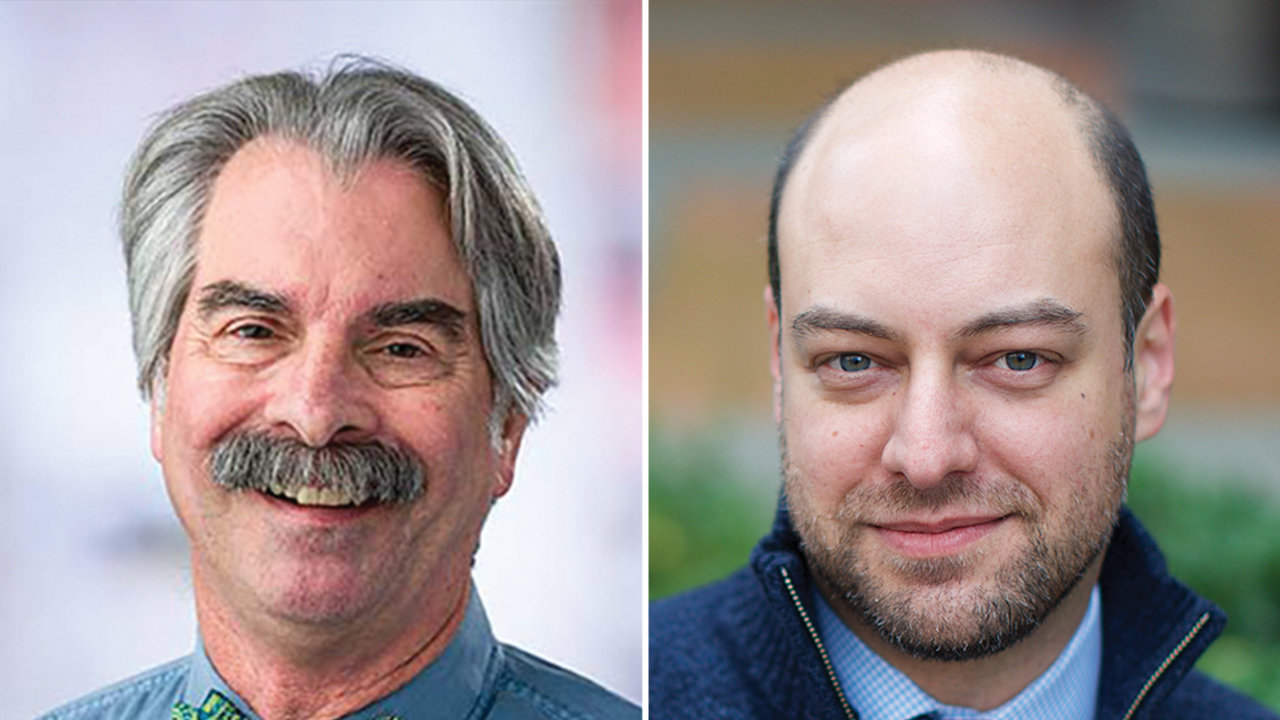
While many patients diagnosed with acute myeloid leukemia (AML) respond to initial treatment, relapse remains the major obstacle to cure. The application of chemotherapy to relapsed AML is unlikely to yield long-term responses, so alternative modalities are sorely needed. Hematopoietic stem cell transplant (HSCT), the only curative therapy for relapsed AML, relies on the combination of high-dose chemotherapy and the graft-versus-leukemia (GVL) effect. T-cell activation is a critical feature of GVL and appears to restore long-lasting tumor immune surveillance in a variety of otherwise fatal hematologic malignancies. Unfortunately, the often short-term clinical activity observed in trials of T-cell-activating therapies suggests that T-cell activation alone is insufficient to recreate the lasting antitumor immunity that characterizes successful GVL.
A growing number of studies suggest the inflammatory milieu created by AML drives AML progression and directly limits T-cell antitumor reactivity. This new understanding is driving the development of a new generation of immunotherapies that incorporates “inflamodulation” to combat or reverse the immunomodulatory effects of the AML inflammatory environment.
The Critical Role of T Cells in GVL
The foundational role T cells play in support of the clinical activity of HSCT is clear.1 The additional infusion of T cells in the context of donor lymphocyte infusion can rescue failed HSCTs, while depleting grafts of T cells increases the risk of relapse.2 These data are buttressed by the ability to isolate donor-derived, leukemia-reactive, activated cytotoxic T lymphocytes from patients after HSCT capable of eliminating leukemic stem cells.3,4 Together, these experiments suggested that if only T cells could be sufficiently activated, anti-AML tumor surveillance could be restored and lead to durable cures without the side effects of transplant. Efforts to engineer leukemia-specific T-cell activation were attempts to make good on this promise.
The immune environment of AML opposes these efforts. At diagnosis and relapse, endogenous T cells within patients with AML are reactive to tumor antigens but exert ineffective immune responses.5 In some ways, this is tautology: if immune surveillance were effective, AML would not manifest in the first place. Nonetheless, identification of leukemia-reactive T-cell receptor (TCR) clones6-8 and high-dimensional mapping of T-cell states9,10 from leukemic and post-treatment bone marrow samples has allowed the identification of mechanisms that stifle effective immune responses. More broadly, transcriptional profiling of large patient cohorts suggests that approximately 25% of patients with AML exhibit immune states that modulate patient outcomes.11-14 These include proliferation of regulatory T cells15 and accumulation of exhausted16-18 or anergic19 cytotoxic T lymphocytes, some of which may be reversible,5 suggesting an avenue to therapeutic intervention. Efforts to modulate this immune inflammatory environment for therapeutic effect are called “inflamodulation.”
Three approaches to synthetic leukemia-specific T-cell activation in patients are now maturing: (1) multispecific T-cell-activating antibodies, (2) transgenic delivery of engineered TCRs and chimeric antigen receptors (CARs), and (3) immune checkpoint blockade. The first two of these technologies share the strategy of providing synthetic antigenic signaling, while the third removes negative signals that suppress responses already present.
The clinical experience of flotetuzumab (MGD006) in a phase I/II trial supports the claim that T-cell activation alone is insufficient to induce lasting remissions in most patients.20 While expression of CD123 was required for trial entry, the overall rate of complete remission (CR) or CR with incomplete hematologic recovery was only 18% following treatment with an optimized drug dosing scheme. However, remission was more likely in patients with bone marrow transcriptional gene signatures associated with neutrophil and macrophage infiltration and interferon (IFN)-γ production, even in patients with the usually dire TP53 mutations.21 These data support that combining T-cell activation with the proper inflammatory milieu is a requirement for producing the long-term remissions observed following transplant with hoped-for frequencies.
Evaluation of CARs targeting CD33,22,23 CD123,24,25 CLL1,26 CD7,27 FLT3,28 and other antigens seems to further undermine claims that the root cause of failure is the lack of a suitable target antigen. Constructs targeting each of these antigens produce T-cell activation in vitro and in preclinical models but have not yet demonstrated clinical activity. As a result, it seems that only B-cell malignancies, including lymphocytic leukemia, lymphomas, and myeloma exhibit the proper environment to sustain long-term tumor immune surveillance following synthetic T-cell activation.
New Approaches to Immune-Environmental Engineering
“Immunotherapists” have recognized that T-cell activation alone is insufficient to overcome the powerful immune-suppressive effects of AML. As a result, novel classes of immune-active therapies that attempt to either reverse the effects of the inflammatory AML microenvironment or supply missing signals that sustain T-cell survival and antitumor activity are being evaluated.
Inflamodulation is increasingly recognized as a successful strategy for treating myeloproliferative neoplasms. The high frequency of JAK2 mutations and the clinical activity of JAK 2 inhibitors have led some investigators to explore combinations of JAK2 inhibition with AML-type therapies. Small studies have found the doublet combination of ruxolitinib with hypomethylating agents is seemingly rarely effective for the treatment of relapsed or refractory patients.29,30 Nonetheless, while JAK2 mutations are infrequent among patients with AML, activation of down stream JAK2 targets, such as STAT3, is common.31 Thus, more specific and potent T-cell-activating strategies will be needed.
Super-charged T cells are coming, and the modularity of CAR designs leads these adoptive cell therapies to the forefront of inflamodulatory testing. Incorporating a secreted anti-IL6 antibody into the CAR design not only eliminated neurotoxicity and made cytokine release syndrome a rare or limited event, but also maintained high response rates (90% among the acute lymphocytic leukemia cohort).32 Incorporation of secreted IL15 may provide both pro-survival and anti-immunosuppressive signals, thereby reversing the effects of myeloid-derived suppressor cells,33 which are increased in AML.34 Secreted IL15,35 membrane-bound IL15,36 and injected IL15-polymer conjugates37 are therefore being explored.
While cytokines create a pro-survival milieu, a super-charged receptor “engine” may break through environmental limitations of T-cell responses. Traditional CAR designs contain CD3 and 4-1BB signaling domains, and “strapping on” additional signaling domains like CD40 may boost antigenic signals, while activation of MYD88 may favorably tune metabolism.38 The number of these studies and their rapid pace suggest CAR technology is an ideal model and platform for evaluating the signals needed to generate lasting GVL.
Attempts to simultaneously limit inflammation and enhance T-cell reactivity may be generalized through modulation of IFNγ. In the case of flotetuzumab, IFNγ production is a strong marker of T-cell activity and balances antihost with antileukemic allo-reactivity;39,40 therefore, modulating IFNγ is a promising strategy to boost GVL and antileukemic responses.41 Boosting IL18 signaling is one novel and promising strategy. A potent soluble inducer of IFNγ, IL18 activity can be supplemented by providing a synthetic decoy-resistant IL18 (DR18),42 while simultaneously removing exhausted T cells via administration of cyclophosphamide following haploidentical transplants43 or providing memory cells through infusion of donor lymphocytes. It is uncertain whether this strategy seeking to supercharge GVL can be translated to clinical testing without undue toxicity.
The Best Weed Suppression Is a Healthy Crop
The inflammatory environment produced by AML not only limits T-cell antitumor surveillance but also drives disease progression. Working in zebrafish models of clonal hematopoiesis of indeterminant potential (CHIP), the laboratory of Lenard Zon44 showed that inflammatory signals elaborated by CHIP clones limited the growth of normal hematopoietic stem cells (HSCs). Genetic evaluation of preleukemic CHIP clones revealed evolved resistance to inflammatory signals. These findings suggested a positive feedback loop in which preleukemic clones and leukemic stem cells are simultaneously a source of and resistant to inflammatory signals, setting up an environment that maintains an advantage for AML cells over residual normal HSCs.
Expression of genes responsive to inflammatory signaling is predictive of chemotherapy resistance45 and improves risk stratification.46 Nearly half of AMLs are enriched in expression of genes associated with inflammation.47 In one study, mechanistic interrogations using short hairpin RNA (shRNA) screens found that mediators of inflammatory signaling were standout targets,47 with hits converging on MYB and SPI/PU.1, which function as “master switches” linking monocytic differentiation with inflammatory signaling.48 Additional shRNA screens also targeted an unstudied gene, IRF2BP2, which, upon investigation, similarly functions to repress inflammatory signaling. Findings that IRF2BP2 knockdown restored sensitivity to TNFα and IL1β and promoted AML apoptosis confirm the generalized model and have since been supported by a growing number of studies, wherein resistance to inflammatory signals is a critical component of the AML microenvironment.
Attempts to synthetically engineer the positive lasting immune activation seen during GVL following HSCT without graft-versus-host disease is a worthy, if incompletely realized, goal. Is therapeutic modulation of the AML inflammatory microenvironment the missing key to engineering GVL-like AML responses? Such “inflamodulation” is an underexplored area of clinical research and tempting to pursue. The next wave of immunotherapies will be the first to explore applications of this new understanding.
Jerald Radich, MD, is a Professor in the Clinical Research Division and Kurt Enslein Endowed Chair at Fred Hutch.
Jacob Appelbaum, MD, PhD, is an Acting Instructor in the Division of Hematology at the University of Washington School of Medicine.
References
- Biernacki MA, Sheth VS, Bleakley M. T cell optimization for graft-versus-leukemia responses. JCI Insight. 2020. doi:10.1172/jci.insight.134939
- Horowitz MM, Gale RP, Sondel PM, et al. Graft-versus-leukemia reactions after bone marrow transplantation. Blood. 1990;75(3):555-562. PMID:2297567
- Chapuis AG, Egan DN, Bar M, et al. T cell receptor gene therapy targeting WT1 prevents acute myeloid leukemia relapse post-transplant. Nat Med. 2019;25(7):1064-1072. doi:10.1038/s41591-019-0472-9
- Chapuis AG, Ragnarsson GB, Nguyen HN, et al. Transferred WT1-reactive CD8+ T cells can mediate antileukemic activity and persist in post-transplant patients. Sci Transl Med. 2013. doi:10.1126/scitranslmed.3004916
- Lamble AJ, Kosaka Y, Laderas T, et al. Reversible suppression of T cell function in the bone marrow microenvironment of acute myeloid leukemia. Proc Natl Acad Sci U S A. 2020;117(25):14331-14341. doi:10.1073/pnas.1916206117
- Montagna D, Maccario R, Montini E, et al. Generation and ex vivo expansion of cytotoxic T lymphocytes directed toward different types of leukemia or myelodysplastic cells using both HLA-matched and partially matched donors. Exp Hematol. 2003;31(11):1031-1038. doi:10.1016/s0301-472x(03)00230-3
- Montagna D, Maccario R, Locatelli F, et al. Emergence of antitumor cytolytic T cells is associated with maintenance of hematologic remission in children with acute myeloid leukemia. Blood. 2006;108(12):3843-3850. doi:10.1182/blood-2006-05-021535
- Graf C, Heidel F, Tenzer S, et al. A neoepitope generated by an FLT3 internal tandem duplication (FLT3-ITD) is recognized by leukemia-reactive autologous CD8+ T cells. Blood. 2007;109(7):2985-2988. doi:10.1182/blood-2006-07-032839
- Sayitoglu EC, Luca BA, Boss AP, et al. AML/T cell interactomics uncover correlates of patient outcomes and the key role of ICAM1 in T cell killing of AML. bioRxiv. 2023. doi:10.1101/2023.09.21.558911
- Abbas HA, Hao D, Tomczak K, et al. Single cell T cell landscape and T cell receptor repertoire profiling of AML in context of PD-1 blockade therapy. Nat Commun. 2021;12(1):6071. doi:10.1038/s41467-021-26282-z
- Rutella S, Vadakekolathu J, Mazziotta F, et al. Immune dysfunction signatures predict outcomes and define checkpoint blockade-unresponsive microenvironments in acute myeloid leukemia. J Clin Invest. 2022. doi:10.1172/JCI159579
- Stratmann S, Yones SA, Garbulowski M, et al. Transcriptomic analysis reveals proinflammatory signatures associated with acute myeloid leukemia progression. Blood Adv. 2022;6(1):152-164. doi:10.1182/bloodadvances.2021004962
- Docking TR, Parker JDK, Jädersten M, et al. A clinical transcriptome approach to patient stratification and therapy selection in acute myeloid leukemia. Nat Commun. 2021;12:2474. doi:10.1038/s41467-021-22625-y
- Cheng WY, Li JF, Zhu YM, et al. Transcriptome-based molecular subtypes and differentiation hierarchies improve the classification framework of acute myeloid leukemia. Proc Natl Acad Sci U S A. 2022. doi:10.1073/pnas.2211429119
- Kanakry CG, Hess AD, Gocke CD, et al. Early lymphocyte recovery after intensive timed sequential chemotherapy for acute myelogenous leukemia: peripheral oligoclonal expansion of regulatory T cells. Blood. 2011;117(2):608-617. doi:10.1182/blood-2010-04-277939
- Knaus HA, Berglund S, Hackl H, et al. Signatures of CD8+ T cell dysfunction in AML patients and their reversibility with response to chemotherapy. JCI Insight. 2018. doi:10.1172/jci.insight.120974
- Noviello M, Manfredi F, Ruggiero E, et al. Bone marrow central memory and memory stem T-cell exhaustion in AML patients relapsing after HSCT. Nat Commun. 2019;10(1):1065. doi:10.1038/s41467-019-08871-1
- Toffalori C, Zito L, Gambacorta V, et al. Immune signature drives leukemia escape and relapse after hematopoietic cell transplantation. Nat Med. 2019;25(4):603-611. doi:10.1038/s41591-019-0400-z
- Narita M, Takahashi M, Liu A, et al. Leukemia blast-induced T-cell anergy demonstrated by leukemia-derived dendritic cells in acute myelogenous leukemia. Exp Hematol. 2001;29(6):709-719. doi:10.1016/s0301-472x(01)00636-1
- Uy GL, Aldoss I, Foster MC, et al. Flotetuzumab as salvage immunotherapy for refractory acute myeloid leukemia. Blood. 2021;137(6):751-762. doi:10.1182/blood.2020007732
- Vadakekolathu J, Lai C, Reeder S, et al. TP53 abnormalities correlate with immune infiltration and associate with response to flotetuzumab immunotherapy in AML. Blood Adv. 2020;4(20):5011-5024. doi:10.1182/bloodadvances.2020002512
- Kenderian SS, Ruella M, Shestova O, et al. CD33-specific chimeric antigen receptor T cells exhibit potent preclinical activity against human acute myeloid leukemia. Leukemia. 2015;29(8):1637-1647. doi:10.1038/leu.2015.52
- Rafiq S, Purdon TJ, Schultz LM, Brentjens RJ. CD33-directed chimeric antigen receptor (CAR) T cells for the treatment of acute myeloid leukemia (AML). Blood. 2016;128:2825-2825. doi:10.1182/blood.V128.22.2825.2825
- Budde L, Song JY, Kim Y, et al. Remissions of acute myeloid leukemia and blastic plasmacytoid dendritic cell neoplasm following treatment with CD123-specific CAR T cells: a first-in-human clinical trial. Blood. 2017;30(Supplement 1):811. doi:10.1182/blood.V130.Suppl_1.811.811
- Cai T, Galetto R, Gouble R, et al. Pre-clinical studies of anti-CD123 CAR-T cells for the treatment of blastic plasmacytoid dendritic cell neoplasm (BPDCN). Blood. 2016;128(22):4039. doi:10.1182/blood.V128.22.4039.4039
- Liu F, Cao Y, Pinz K, et al. First-in-human CLL1-CD33 compound CAR T cell therapy induces complete remission in patients with refractory acute myeloid leukemia: update on phase 1 clinical trial. Blood. 2018;132(Supplement 1):901. doi:10.1182/blood-2018-99-110579
- Gomes-Silva D, Atilla E, Atilla PA, et al. CD7 CAR T cells for the therapy of acute myeloid leukemia. Mol Ther. 2019;27(1):272-280. doi:10.1016/j.ymthe.2018.10.001
- Jetani H, Garcia-Cadenas I, Nerreter T, et al. CAR T-cells targeting FLT3 have potent activity against FLT3-ITD+ AML and act synergistically with the FLT3-inhibitor crenolanib. Leukemia. 2018;32(5):1168-1179. doi:10.1038/s41375-018-0009-0
- Pemmaraju N, Kantarjian H, Kadia T, et al. A phase I/II study of the Janus kinase (JAK)1 and 2 inhibitor ruxolitinib in patients with relapsed or refractory acute myeloid leukemia. Clin Lymphoma Myeloma Leuk. 2015;15(3):171-176. doi:10.1016/j.clml.2014.08.003
- Bose P, Verstovsek S, Cortes JE, et al. A phase 1/2 study of ruxolitinib and decitabine in patients with post-myeloproliferative neoplasm acute myeloid leukemia. Leukemia. 2020;34(9):2489-2492. doi:10.1038/s41375-020-0778-0
- Steensma DP, McClure RF, Karp JE, et al. JAK2 V617F is a rare finding in de novo acute myeloid leukemia, but STAT3 activation is common and remains unexplained. Leukemia. 2006;20(6):971-978. doi:10.1038/sj.leu.2404206
- Xue L, Yi Y, Xu Q, et al. Chimeric antigen receptor T cells self-neutralizing IL6 storm in patients with hematologic malignancy. Cell Discov. 2021;7(1):84. doi:10.1038/s41421-021-00299-6
- Zannikou M, Duffy JT, Levine RN, et al. IL15 modification enables CAR T cells to act as a dual targeting agent against tumor cells and myeloid-derived suppressor cells in GBM.
J Immunother Cancer. 2023. doi:10.1136/jitc-2022-006239 - Pyzer AR, Stroopinsky D, Rajabi H, et al. MUC1-mediated induction of myeloid-derived suppressor cells in patients with acute myeloid leukemia. Blood. 2017;129(13):1791-1801. doi:10.1182/blood-2016-07-730614
- Zhang Y, Zhuang Q, Wang F, et al. Co-expression IL-15 receptor alpha with IL-15 reduces toxicity via limiting IL-15 systemic exposure during CAR-T immunotherapy. J Transl Med. 2022;20(1):432. doi:10.1186/s12967-022-03626-x
- Sun Y, Su Y, Wang Y, et al. CD19 CAR-T cells with membrane-bound IL-15 for B-cell acute lymphoblastic leukemia after failure of CD19 and CD22 CAR-T cells: case report. Front Immunol. 2021. doi:10.3389/fimmu.2021.728962
- Hirayama AV, Chou CK, Miyazaki T, et al. A novel polymer-conjugated human IL-15 improves efficacy of CD19-targeted CAR T-cell immunotherapy. Blood Adv. 2023;7(11):2479-2493. doi:10.1182/bloodadvances.2022008697
- Mata M, Gerken C, Nguyen P, Krenciute G, Spencer DM, Gottschalk S. Inducible activation of MyD88 and CD40 in CAR T cells results in controllable and potent antitumor activity in preclinical solid tumor models. Cancer Discov. 2017;7(11):1306-1319. doi:10.1158/2159-8290.CD-17-0263
- Yang YG, Qi J, Wang MG, Sykes M. Donor-derived interferon gamma separates graft-versus-leukemia effects and graft-versus-host disease induced by donor CD8 T cells. Blood. 2002;99(11):4207-4215. doi:10.1182/blood.v99.11.4207
- Wang H, Yang YG. The complex and central role of interferon-γ in graft-versus-host disease and graft-versus-tumor activity. Immunol Rev. 2014;258(1):30-44. doi:10.1111/imr.12151
- Lu Y, Waller EK. Dichotomous role of interferon-gamma
in allogeneic bone marrow transplant. Biol Blood
Marrow Transplant. 2009;15(11):1347-1353. doi:10.1016/
j.bbmt.2009.07.015 - Zhou T, Damsky W, Weizman OE, et al. IL-18BP is a secreted immune checkpoint and barrier to IL-18 immunotherapy. Nature. 2020;583(7817):609-614. doi:10.1038/s41586-020-2422-6
- Minnie SA, Waltner OG, Ensbey KS, et al. Depletion of exhausted alloreactive T cells enables targeting of stem-like memory T cells to generate tumor-specific immunity. Sci Immunol. 2022. doi:10.1126/sciimmunol.abo3420
- Avagyan S, Henninger JE, Mannherz WP, et al. Resistance to inflammation underlies enhanced fitness in clonal hematopoiesis. Science. 2021;374(6568):768-772. doi:10.1126/science.aba9304
- Vadakekolathu J, Minden MD, Hood T, et al. Immune landscapes predict chemotherapy resistance and immunotherapy response in acute myeloid leukemia. Sci Transl Med. 2020. doi:10.1126/scitranslmed.aaz0463
- Lasry A, Nadorp B, Fornerod M, et al. An inflammatory state remodels the immune microenvironment and improves risk stratification in acute myeloid leukemia. Nat Cancer. 2023;4(1):27-42. Published correction appears in Nat Cancer. 2023;4(1):149. doi:10.1038/s43018-023-00518-x
- Ellegast JM, Alexe G, Hamze A, et al. Unleashing cell-intrinsic inflammation as a strategy to kill AML blasts. Cancer Discov. 2022;12(7):1760-1781. doi:10.1158/2159-8290.CD-21-0956
- Chavez JS, Rabe JL, Loeffler D, et al. PU.1 enforces quiescence and limits hematopoietic stem cell expansion during inflammatory stress. J Exp Med. 2021. doi:10.1084/jem.20201169